Posted in:
Celebrating 40 Years of the Oceanic Flux Program
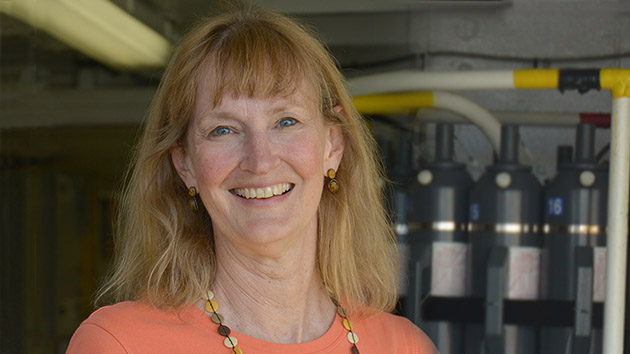
BIOS faculty member Maureen Conte, an organic geochemist, has led the OFP time-series for nearly 25 years. “In the 1960s, people thought the Sargasso Sea was an uninteresting place,” she said. “We’ve shown that, in fact, it is quite the opposite.”
In April, the Oceanic Flux Program (OFP), the longest running deep ocean time-series of its kind, marked its 40th year of operation. Since 1978, the OFP’s three sediment traps have continuously sampled sinking particulate debris, called particle flux, at depths of 1,640; 4,900; and 10,500 feet (500; 1,500; and 3,200 meters) at a location about 45 miles (75 kilometers) southeast of Bermuda.
The deep ocean particle flux consists of a mixture of settling particles comprising organic and skeletal remains of plankton, as well as suspended particles that become incorporated into sinking organic debris. These include land-derived materials that are transported into ocean gyres by currents or winds; minerals precipitated within the water column; and dissolved compounds that become adhered to particle surfaces.
BIOS faculty member Maureen Conte, an organic geochemist, has led the OFP time-series for nearly 25 years, taking over in 1994 from its founder Werner Deuser, a geochemist from Woods Hole Oceanographic Institution.
“So much has changed about the technology we use—it drives the discovery,” Conte said. “Everything has improved,” she said, from the size and programmability of the traps, to the sophistication of the moored instrumentation, to the trace level analytical chemical techniques used to analyze the recovered flux materials. Yet, throughout the changes of the last 40 years, an OFP sediment trap has sampled the deep particle flux at this site in the Sargasso Sea.
Why study this area of the ocean?
Conte: The OFP site is located in the northern Sargasso Sea in the western part of the North Atlantic gyre. This area is typical of the low-productivity, low-nutrient ocean regions that comprise about 90 percent of the total ocean area, which means that this spot provides an excellent snapshot of a much larger ocean area.
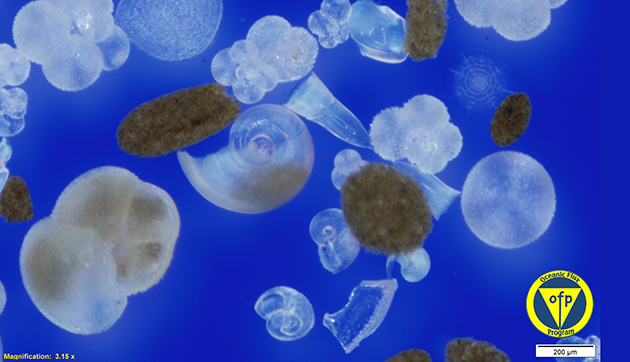
The sediment traps on the OFP mooring collect a variety of biological materials that contribute to the sinking flux. Pictured here is an image of a variety of shells of microscopic foraminifera and pteropod species and zooplankton fecal pellets in the deep ocean particle flux at 10,500 feet (3200 m) depth.
…and why study the deep particle flux?
We study the flux because sinking particles carry with them many different elements and organic compounds. Some elements in the sinking flux are essential to support life. The depths at which these are dissolved and then redistributed by ocean currents (and rise again to the surface) control nutrient distributions that regulate the ocean’s productivity, the global cycles of many elements, and the rate at which the ocean can absorb carbon dioxide from the atmosphere. Sinking organic debris, and what is left behind by migrating zooplankton, provides the food which fuels most life below the sunlit surface of the ocean. The residual material that survives to be deposited on the seafloor and preserved in the sediments retains a rich record of past ocean conditions that is used to reconstruct Earth’s history.
How exactly do you measure the particle flux?
We use a deep ocean subsurface mooring that is anchored in 2.7 miles (4,500 meters) of water and extends to 984 feet (300 meters) below the surface. The wire rope mooring line has floatation attached to keep it vertical, sediment traps to collect the particle flux, and specialized instruments to measure physical properties, such as water temperature, as well as chemical properties, like salinity and oxygen, of the seawater. At the top of the mooring, an instrument called an upward-looking Acoustic Doppler Current Profiler (ADCP) continuously profiles the speed and direction of currents in the overlying water column up to the surface. Acoustic releases are attached near the bottom of the mooring, at 13,000 feet (4,000 meters) depth.
We arrive at the mooring site by relying on the ship’s GPS navigation to locate its position. Then we communicate with the releases using a special acoustic transducer mounted below the ship. We send a coded signal telling one release to activate a pin, which drops the anchor and allows the mooring to rise to the surface.
When it reaches the surface, a radio, flasher and Iridium satellite transmitter mounted on the ADCP buoy begin signaling so we can locate the mooring and begin recovery operations. During recovery, we retrieve the sample bottles on the sediment traps, download data, service the equipment, reload fresh trap bottles, reprogram instruments, and replace mooring components, if needed. By the next day the mooring is ready to be redeployed, descending again through the water column to begin sampling the deep particle flux that night, a total sampling hiatus of only 48 hours.
What’s been most compelling to you during your years at the helm of the OFP?
Contrary to what we thought 40 years ago, the deep ocean environment is really quite sensitive to what’s happening at the surface and within the ocean interior. The deep flux is driven by surface biology and physics, but it is also tightly coupled to the activities of the deep ocean ecosystems which recycle and modify particles within the water column. We also have a greater appreciation for the importance of short-lived events, such as blooms induced by ocean eddies and extreme weather like storms and hurricanes, which enhance the transport of fresh organic material from the surface to the deep ocean. The implication is that changes to the surface ocean, in relation to climate change, are going to be transferred to the deep ocean.
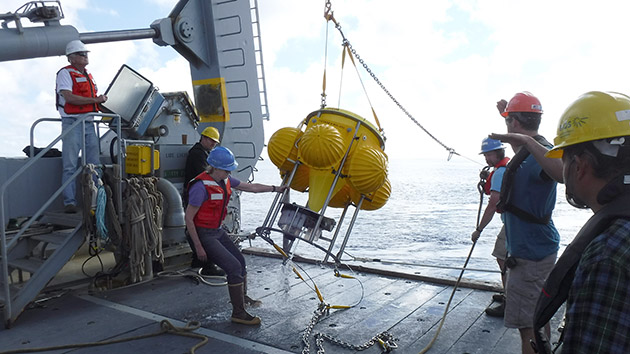
Conte (middle) and fellow scientists and crew members recover the mooring at the OFP site offshore Bermuda. The subsurface mooring is anchored in 2.7 miles (4,500 meters) of water and extends to 984 feet (300 meters) below the surface.
The OFP’s sample archive is a unique treasure and we’re making made great strides in applying sophisticated chemical methods to probe the information it contains about how the ocean has changed over the past 40 years. For example, we’ve been collaborating with BIOS scientist Amy Maas and others in studying the influences of climate variability and ocean acidification on pteropod species, which are important members of the zooplankton community. Their shells are major components of the inorganic carbon flux that controls the strength of the ocean’s uptake of carbon dioxide.
You received updates on your phone from the mooring via satellite this summer, just before the start of a hiking vacation in Wyoming, and you learned that the mooring was floating free from its anchor. What happened?
In late August, the OFP mooring parted from the anchor due to a defective stainless steel mooring chain. We found out when we removed the mooring that the chain was a fake; it was coated in stainless steel but the middle of it basically looked like oatmeal.
Fortunately, the Iridium satellite transmitter on the top of the mooring worked flawlessly and sent signals alerting my research team to the unexpected surfacing. My team immediately began arranging an emergency research cruise to recover the wayward mooring. I am grateful to crew members of the BIOS research vessel Atlantic Explorer, including marine superintendent Quentin Lewis and captain George Gunther, for quickly mounting this emergency recovery cruise, and to BIOS staff members Matt Hayden and Fernando Pacheco who assisted with the recovery and redeployment operations.
What’s the future of the program?
We are going to keep on doing what we’ve been good at doing: providing a high quality, continuous record of the deep particle flux. But we’ll keep doing it in better ways. The OFP mooring will contain more sophisticated instruments to provide essential data on oceanographic properties. The flux record itself will also be increasingly valuable by the data provided by other local time-series, Hydrostation S and BATS, gliders and other studies that are gathering more details about this area of the ocean.
We’ll be applying advances in microscopy to improve the digital image archive we generate on the larger particle fractions that provide a unique multi-dimensional view of the dynamics of ocean particle composition. These images can now be accessed and analyzed by researchers around the world, without ever touching a sample. We’re working right now with data specialists to improve ways of organizing these data streams for people to access remotely. There will be continuing advances in analytical capabilities; the trace level organic and elemental analyses we routinely make on samples today couldn’t have been imagined when the OFP began. It’s fun to ponder what information we’ll be able to extract from the samples when the OFP celebrates its 50th anniversary!
In the 1960s, people thought the Sargasso Sea was an uninteresting place. We’ve shown that, in fact, it is quite the opposite; it’s a place of dynamic, seasonal changes. The amount of information we’ve learned about the particle flux and how it is coupling with ocean ecosystems and ultimately our climate would be unbelievable to people doing this research 40 years ago, and it continues to thrill me to envision what more we’ll discover.
Tagged: